I have been seeing a lot of speculation lately about how space mining will solve the critical mineral shortages that will be coming in the future. All of the talk about mining the moon, Mars and the Asteroid Belt strikes me as the ludicrous fantasies of people who don’t want to accept the resource limits that humanity faces.
It is understandable why people see space mining as a solution, as they survey the growing demand for minerals. According the US Geological Survey, the world mined 26 megatons of copper in 2022, but only has 890 megatons of copper reserves. Assuming that no more reserves are found, at the current rate of consumption, we will run out of copper in 34.2 years. Rising prices and new extraction techniques will make it possible to mine copper in more places on the planet, but we will also need massive amounts of copper to switch the world from running on fossil fuels to renewable energy. Today’s electric vehicles contain 3 times more copper than internal combustion engine vehicles, so futurists worry that lots more copper will be needed to electrify all transport.
The world won’t run out of copper in the next 3 to 4 decades, but it will get much harder to extract, as mining companies have to switch from sulfuride ores to laterite ores, which means moving a lot more earth and consuming a lot more energy to extract the same amount of copper. Mining will have to move to lower grades of ores with lower concentrations of copper and operate in more remote locations such as the ocean floor.
The world is going to face shortages of many of the critical minerals in the future. With current extraction rates and today’s reserves, the world only has 14.8 years of tin, 16.2 years of zinc, 16.8 years of gold, 21.2 years of silver, 30.3 years of nickel, 43.7 years of cobalt and 48.0 years of molybdenum. There is plenty of tin and zinc in the Earth’s crust, but they previously weren’t considered economically feasible to extract so they weren’t listed as reserves. With higher prices in the future caused by shortages, they will become extractable. However, there are higher costs to extracting from these new reserves since their ore often has low concentrations of the metal, so it requires moving more earth in the mining and using more energy in the refining, so it is more polluting to extract the same amount of metal.
Rather than pollute the planet with all this new mining, it is tempting to look to outer space to solve our resource limits. The average metallic asteroid holds a concentration of iron, nickel, cobalt and platinum which is 22, 1163, 300 and 29 times higher than in the Earth’s crust, respectively. A NASA study estimates that the Asteroid Belt holds $700 quintrillion in mineral wealth, which would be $85 billion for each inhabitant on Earth. Of course if all those minerals were brought back to Earth, they would cause a total collapse in the price of platinum, gold, nickel, cobalt, ruthenium, rhodium, palladium, osmium, iridium and many other metals. The asteroids with the most potential value are carbonaceous chondrites, such as Davida, which is roughly 300 km in diameter and is estimated to hold $27 quintrillion in mineral wealth, with a rich supply of iron, nickel, cobalt, platinum, nitrogen, ammonia and hydrogen. Asterank, which is a database of 600,000 asteroids, estimates that mining Davida would generate over 100 trillion in profits.
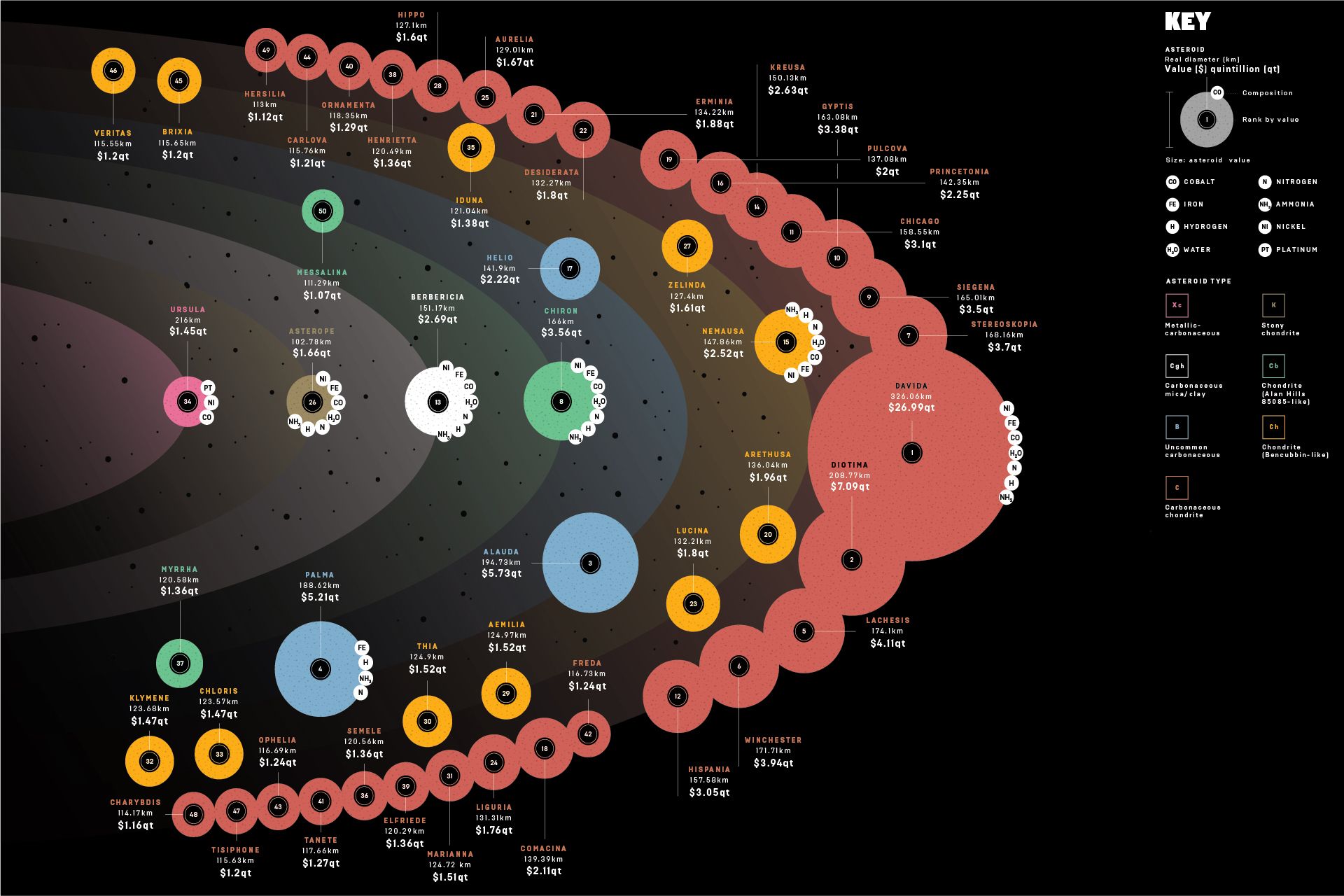
The first generation of space mining firms, such as Deep Space Industries and Planetary Resources were formed in the 2010s. Both companies identified about half a dozen promising asteroids in the inner Asteroid Belt with mining potential, but Deep Space Industries and Planetary Resources went belly up in 2018 and 2019, respectively, due to lack of funding. New firms have sprung up in the last two years, such as AstroForge, TransAstra and Karman+, which also hope to extract the potential mineral wealth in outer space. This second generation of space mining companies will likely run into the same problems as the first generation, because it is going to be many decades before space mining is feasible.
A lot of new technology needs to developed in order to mine asteroids, such as robotic mining machines with artificial intelligence that can work remotely without direct human supervision. There are huge logistical challenges to getting all that mining equipment onto a tiny asteroid located between 478.7 to 628.3 million km from Earth. NASA thinks that one of its Artemis moon landings will require up to 20 refueling launches for SpaceX’s enormous Starship, so an asteroid mining operation would probably require hundreds of refueling launches in order to get all the equipment to the asteroid and haul back the minerals. They can find water on the moon, Mars and many of the asteroids, but that means doing water mining and setting up an expensive electrolysis station to split the water into oxygen and hydrogen to fuel the trip back to Earth. A mining operation will probably need to use a methane rocket since it is hard to make reusable hydrogen rockets due to the problem of hydrogen embrittlement of the metal. Therefore, carbon needs to be extracted from the soil (or from the atmosphere on Mars) and then joined to hydrogen to create CH4.
I doubt that space mining is going to be technically feasible before the 2050s. Even when it becomes possible, I doubt that it will ever be economically viable for most of the minerals which are going to face shortages in the future.
The cost of getting a kg to low Earth orbit (LEO) has been reduced from $54,500 with the Space Shuttle to $1500 with SpaceX’s Falcon Heavy, and the upcoming Starship promises to take that cost down to $200. Let’s say that we can get launch costs to LEO down to $50 per kg by the 2050s. We will still have to travel to the asteroid belt to do the mining and haul the minerals back to Earth, which will probably cost between $10,000 and 20,000 per kg in the beginning. With enough scale, maybe we can get that cost down to $500-$1000 per kg. Those costs might work for precious metals, but I can’t see it ever working for critical metals like copper and nickel, that will be suffering shortages by mid-century.
In addition, we don’t know what will be the climatic and ozone impacts of all the rocket launches that will be needed for space mining. Martin Ross, who has been researching this problem over the last two decades, believes that the growth in rocket emissions will deplete the ozone layer, alter jet streams and change global circulation patterns. A recent study by NOAA and Ross calculates that a 10 times increase in current soot emissions from rocket launches will have a significant impact on temperatures in the stratosphere and slow down atmospheric jet streams.
The chlorine and alumina particles emitted by solid rocket fuels destroy ozone (O3), which protects life on Earth. Ozone absorbs the dangerous ultraviolet B radiation from the sun with a wavelength between 280 and 320 nanometer, which damages DNA, causes skin cancer and cataracts (clouding of the eye lens), damages the growth of plants, reduces the survival rates of phytoplankton and harms the reproduction and larval development of fish, shrimp, crab, amphibians, and other marine animals. The black carbon (i.e. soot), which is produced by the incomplete combustion of fossil fuels, absorbs sunlight and heats the stratosphere, which in turn accelerates the chemical reactions which destroy ozone. Alumina particles stay for 1 to 2 years in the stratosphere and black carbon particles stay for 3 or 4 years in the stratosphere, so the effects of rocket emissions are long lasting.
The amount of CO2 produced by current rocket emissions is not very significant, but future rocket launches may have a significant impact on the amount of water vapor in the stratosphere, which could alter the temperature of the stratosphere. A molecule of water stays an average of 8.9 days in the troposphere, but it stays for months in the stratosphere, where it traps heat. Hydrogen rockets emit water vapor directly into the stratosphere. Methane (CH4) rockets burn fuel rich, so not all the methane is combusted. That excess methane will oxidize which generates the HOx radicals which catalytically destroy ozone. In addition, when a methane molecule oxides by combining with two O2 molecules, it produces two H2O and one CO2 molecules, which will trap heat in the stratosphere. The net warming effect of this water vapor and carbon dioxide from a single methane rocket launch is small, but the number of methane rocket launches will likely grow to thousands per year in the future.
The number of orbital rocket launches has increased from 74 in 2010 to 114 in 2020 and to 223 in 2023, so they are growing exponentially. The coming boom in telecommunications and internet satellites, space tourism and plans for space colonization and industrialization promise to massively increase the number of rocket launches. McKinsey predicts that the total amount of orbital payload launched per year will increase from 2 kilotons in 2023 to 40 kilotons in 2030. There are 18 proposed satellite internet constellations which plan to put 537,267 satellites into low Earth orbit. Even though many launch providers are switching from polluting solid fuels and kerosene rockets to cleaner-burning methane rockets, such as SpaceX’s Starship, Blue Origin’s New Gleen, ULA’s Vulcan Centaur, Rocket Lab’s Neutron, Relativity Space’s Terran, Firefly’s Alpha and Landspace’s Zhuque-2, the sheer number these rocket launches are likely to change global temperatures in the stratosphere in the future. We have to ask whether we really need this massive expansion in space entrepreneurialism.
The truth is that we can live within the resource limits of the planet if we prioritize efficient use of resources and recycling, which would be far more sustainable and less costly than mining outer space. We keep figuring out new ways to do more with less resources, so we can live with just the Earth’s existing resources, but we have to chose to make it a priority.
For example, today’s electric vehicles consume a lot of expensive metals (lithium, nickel, cobalt, manganese, copper, aluminum, iron, neodymium, dysprosium, etc.), but the move from NMC to LFP batteries eliminates the nickel, cobalt and manganese. In the future, the move to sodium ion batteries will eliminate the lithium and copper, so EV batteries will only require iron and aluminum which are sustainable metals with nearly limitless reserves.
EVs can be designed with AC induction motors (e.g. Model S/X), wound rotor synchronous motors (e.g. Renault Zoe), switched reluctance motors (e.g. AEM/Bentley) or in-rotor inductive-excited synchronous motors (e.g. ZF) to eliminate the permanent magnets, which contain rare earth elements (REEs) such as neodymium, dysprosium and terbium. Tesla reduced the amount of REEs in its Model 3 drive train by 25% between 2017 and 2022 and promises that its future $25k car will use no REEs in the motor’s permanent magnets, presumably by using ferrite magnets. Tesla’s Cybertruck reduces 50% of the copper in its wiring by using Ethernet+CAN data buses instead of point-to-point wiring harnesses and by using higher voltages (12V -> 48V components and 400V -> 800V batteries/motors), which reduces the thickness of the wires. Wiring and motor coils can also be switched from copper to aluminum in the future if copper becomes too scarce, because there is plenty of bauxite reserves to make aluminum and we can eliminate most of the pollution from smelting by using renewable energy.
The same reduction in resource consumption can happen in the energy sector. Grid batteries are switching from NMC to LFP chemistries and in the future they will use even cheaper types of storage, such as saltwater flow batteries that require no metals except stainless steel in the containers. Today’s wind turbines use a lot less of the critical metals. Onshore wind turbines are switching from permanent magnet direct drives and electrically excited direct drives to geared drives and doubly fed induction generators which use no permanent magnets and much less copper. Offshore wind turbines are switching from permanent magnet direct drives to semi-direct drives, which contain hybrid geared drives and permanent magnet synchronous generators, that only need 18% of the permanent magnets and 22% of the copper used by direct drives. As wind turbines grow in size, they get more efficient and use less copper per kilowatt.
Thin film solar which uses lots of expensive minerals (cadmium, tellurium, copper, indium, gallium and selenium) hasn’t been able to compete with crystalline silicon solar which requires little metal and controls over 90% of the solar market. PV solar cells use expensive silver contact lines, which currently consumes 15% of the world’s silver supply, but many solar manufacturers are now switching to cheaper copper contact lines.
Looking at modern electronics compared to clunky machines of the 1980s, it is amazing how much less material is needed to make today’s electronic devices, and we can reduce the mineral consumption even more if we start focusing on longevity, fixability and recyclability over planned obsolescence. This change is already happening in the phone industry as the standard time that mobile phones are supported with software updates has increased from 2 years to 4 years over the last couple years. The EU has implemented regulations to require that all mobile phones, tablets and cameras use a standard USB-C charging port by the end of 2024 (and laptops by 2026) and all smartphones, tablets, electric bikes and e-scooters contain replaceable batteries by 2027. Likewise the typical lifespan of automobiles has increased from 100,000 to 200,000 miles over the last half century, and EVs with LFP batteries should increase vehicle lifespans in the future to over 400,000 miles.
We can redesign all sorts of things for longevity and fixability, so we need expend less resources in manufacturing throw-away goods. The clothing industry, which produces 8%-10% of the world’s carbon emissions and 20% of the world’s industrial wastewater pollution, has promoted “fast fashion” to shorten the lifespan of clothing. McKinsey estimates that the number of garments purchased per capita increased by 60% between 2000 and 2014, while the average lifespan of garments halved over the same time period. However, there is a growing “slow fashion” movement to go back to making clothes that last longer. Two thirds of the 430 million tons of global plastic produced every year is used in disposable products. We can get rid of most single-use plastics if we charged a tax on single-use bottles, cups and bags and sold things in reusable containers.
The move from fossil fuels to renewable energy means getting rid of wasteful combustion that loses roughly 70% of its energy as heat. An electric motor has energy efficiency between 85% and 95% compared to an internal combustion engine which is 28%-30% efficient. Moving to renewable energy also means eliminating 40% of ocean shipping which is dedicated to hauling fossil fuels around the planet. The move to local agriculture can eliminate another 10% of ocean shipping which is used to carry food around the globe.
Electrifying everything will bring all sorts of new efficiencies and new possibilities. For example, converting to electric heating means a significant reduction in the weight and size of heaters. Heavy ICE scooters can be replaced by electric kick scooters which are a fourth of the weight and can be folded up and carried around. Generating energy from the sun means that all sorts of surfaces can become solar panels, so roofs and outer walls become energy generators. By using aerodynamic designs and stamped carbon fiber bodies, solar cars like the Aptera can be made so energy efficient that they can run on just the energy from their solar panels so that driving become essentially free.
Space mining is just an excuse to continue being wasteful with the Earth’s resources. It is a get out of jail for free card so we aren’t forced to face the hard reality that resources are limited and we need to change how we consume them.